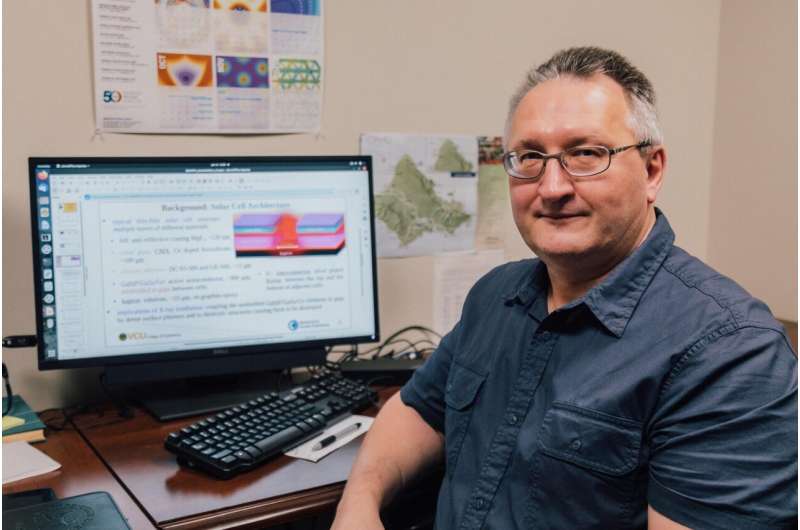
Computational physics is a field of nuance and detail. Using mathematics, researchers build computer models and simulations to test hypotheses within a digital environment. These numerical experiments are often used when practical testing is not feasible such as when, for example, you must ascertain the durability of materials in a nuclear explosion.
Gennady Miloshevsky, Ph.D., is an associate professor of mechanical and nuclear engineering in the Virginia Commonwealth University College of Engineering who specializes in computational physics with an emphasis on plasma, lasers and particle beams. He works to predict the behavior and state of materials when under extreme pressure, temperature and radiation.
With funding from the Defense Threat Reduction Agency, an agency of the U.S. Department of Defense, Miloshevsky is studying the effect weapons of mass destruction have on satellites within Earth’s orbit. His work requires a distinct familiarity with our physical world and how different forms of energy interact with and within matter.
“Any satellite close to the detonation point would be destroyed,” Miloshevsky said. “However, beyond that initial area, surviving satellites could be subject to X-ray-induced blow-off, thermo-mechanical shock and warm dense plasma formation take place on material surfaces. This causes damage to exposed optics, sensors and solar cells on satellites. Particularly dense surface plasmas can couple the solar cells to each other in gaps between unshielded active elements and to dielectric structures causing them to be destroyed. It would all depend on the distance from the detonation point and the orientation of the satellite.”
Part of Miloshevsky’s research involves developing methods to computationally simulate temperature, pressure and radiation in order to study the state known as “warm dense plasma,” which occurs between the solid and classical plasma states and exhibits the characteristics of both. A better understanding of this state of matter is a steppingstone to building more resilient materials.
“Warm dense plasma is highly transient and short lived,” Miloshevsky said. “The state occurs in several nanoseconds, so isolating it in a laboratory setting in order to characterize it is very complicated. A nuclear burst irradiates materials with high-intensity X-rays, resulting in the transition to warm dense plasma. Our DTRA research seeks to understand the fundamental physics of warm dense plasma, including its physical and electrical properties. It’s currently unclear how this may affect the choice of future materials for satellite components.”
A ban on nuclear testing means research into the effects of nuclear weapons is only possible through the use of computer codes to either model or simulate the many physics phenomena generated by a nuclear detonation.
Miloshevsky’s first research area includes quantifying and reducing the uncertainty of computer model material properties, such as diamond, under the conditions of a nuclear blast using REODP (Radiative Emissivity and Opacity of Dense Plasmas) computer code he developed. This code is used to investigate the ionization state and ion abundances for equilibrium and transient-dense plasmas. It helps predict the equations of state, transport and optical properties of materials in the category of warm dense plasma.
In a second research area, Miloshevsky works to understand and predict the interaction between X-rays and satellite surface materials (such as silicon, germanium and other materials used to make solar panels) during a nuclear detonation in space. This uses MIRDIC (Modeling Ionizing Radiation Deep Insulator Charging) code developed in collaboration with NASA’s Marshall Space Flight Center for its Europa Lander project. This code helps anticipate charge production by blackbody X-rays in dielectrics and insulators of DoD space systems. It can also predict electrostatic material breakdown.
Also part of the second research area is work to understand X-ray-induced shock generation, material ablation and blow-off (when material is literally “blown off” the satellite in reaction to another force) within the vacuum of space. This is studied using MSM-LAMMPS (Momentum Scaling Model implemented within the Large-scale Atomic/Molecular Massively Parallel Simulator software package) code. It predicts material behavior at an atomic level within extreme environments, the nature and behavior of materials in highly nonequilibrium states, microscopic mechanisms of disintegration, blow-off, melting, ionization and warm dense plasma states.
Practical experiments in a lab use lasers to replicate the heat and pressure generated by X-ray radiation, shock and other physical effects of a nuclear detonation. Miloshevsky’s colleagues at the John Hopkins Extreme Materials Institute heat carbide diamond and silica materials typically found in solar panels to temperatures between 11,600 and 1,160,000 Kelvin using lasers at the University of Rochester and Pacific Northwest National Laboratory to observe this momentary transformation into warm dense plasma. Researchers use shadowgraphy, spectroscopy and other visual analytical methods to quantify the result. They can also investigate the depth, size and shape of the crater created by the laser within the material surface.
“Experimental and computational research are closely interconnected and complement each other,” Miloshevsky said. “The laser-material interaction is a complicated process that occurs on multiple space (nanometers to millimeters) and time (femtoseconds to milliseconds) scales with evolving and changing physics. Data measured in these experiments usually need physics insights from a computer model to be correctly interpreted and understood. Models can provide fine details of physics processes that cannot be revealed in the practical experiments due to the incredibly minute space and time scales. Conversely, data from physical experimentation can feed into a computer model so it can be further developed and refined to enhance the understanding of the experiment’s measured data.”
Miloshevsky’s recent topical review paper, “Ultrafast Laser Matter Interactions: Modeling Approaches, Challenges, and Prospects,” details some of these advances in computational modeling and simulation development for laser-pulse interactions with solids and plasma. The research is published in Modelling and Simulation in Materials Science and Engineering.
More information:
Gennady Miloshevsky, Ultrafast laser matter interactions: modeling approaches, challenges, and prospects, Modelling and Simulation in Materials Science and Engineering (2022). DOI: 10.1088/1361-651X/ac8abc
Citation:
Researchers strive to predict satellite resilience to weapons of mass destruction in space (2023, February 9)
retrieved 9 February 2023
from https://techxplore.com/news/2023-02-satellite-resilience-weapons-mass-destruction.html
This document is subject to copyright. Apart from any fair dealing for the purpose of private study or research, no
part may be reproduced without the written permission. The content is provided for information purposes only.
Stay connected with us on social media platform for instant update click here to join our Twitter, & Facebook
We are now on Telegram. Click here to join our channel (@TechiUpdate) and stay updated with the latest Technology headlines.
For all the latest Technology News Click Here
For the latest news and updates, follow us on Google News.